Water: Beach Grants
Appendix 4C1: Predictive Tools
This appendix provides supplemental discussions, examples, and additional references that may be helpful to beach program managers. It does not create additional requirements beyond those in the main guidance document.
1. Screening Factors for Model Selection
Selection of an appropriate water quality model for helping to determine beach advisories and closings depends on the site conditions of the waterbody of concern. The selection of the appropriate model can be based on the following screening factors:
- Combined point and nonpoint sources. An important screening factor is how the model handles the loadings from point and nonpoint sources. Models based on water quality data implicitly take the point and nonpoint sources into account, whereas models that use continuous simulation of the water quality directly account for the sources. Typically, the sources are part of the input parameters. For example, the rainfall-based alert curves discussed later in this chapter are models based on water quality conditions. Those models do not explicitly account for point and nonpoint sources; instead, the sources affect the water quality parameters used in the model. In the case of the CORMIX and PLUME models (described below), point sources are a component of the model input; the flow and concentration also must be included.
- Pathogen source characterization. Pathogens found at a beach site of interest might be from point sources, including sewage treatment plants, sanitary sewer overflows (SSOs), combined sewer overflows (CSOs), septic systems, and storm water outfalls, or nonpoint sources. Accounting for the different sources of pathogens might require the use and integration of a variety of models. Once pathogen loads from point and nonpoint sources are determined, the next step is the routing of the pathogen through the system using a representative model of the dominant mixing and transport processes to estimate the pathogen concentration at the location of interest.
- Dominant mixing and transport processes. The waterbody type dictates the dominant mixing and transport processes of a pollutant. In rivers and streams the dominant processes are advection and dispersion. In estuaries these processes are influenced by tidal cycles and flows. Waterbody size and net freshwater flow are also important in determining the dominant processes. For discharges in the ocean surf zone, dominant dispersion processes include mixture due to breaking waves and transport from nearshore currents.
- Pathogen concentration prediction. This factor describes the ability of the model to predict the pathogen concentration in the receiving water at the location of interest, which in this case is a beach site. Transformation processes such as bacterial kinetics must also be accounted for in the model to allow for a realistic prediction.
- Ability to provide time-relevant analysis, decisionmaking, and guideline establishment. Timely or time-relevant analysis is needed for an effective advisory. Models applied to predict water quality conditions can be used as a basis for decision making and as management tools. For example, a beach authority can use such tools as a basis for beach advisories following a rainfall event or an accidental sewage spill.
- Time-relevant use. Under this category the input data needed, processing time, and postprocessing abilities of the model are evaluated. Potential predictive tools for beach advisories must be able to predict pathogen concentration at the site of interest in a relatively short. This means that the data input requirements and processing time need to be minimal. Also crucial to the success of the predictive tool is the postprocessing of the output data. Tabular or graphical representation of the output data provides a quick, easy way to interpret results and might serve as a basis for making time-relevant decisions concerning beach advisories.
- Evaluation of unplanned and localized spills. Spills of a pollutant can be caused accidentally by equipment failure or rainfall. In either case, this factor describes how the model handles the additional loading. Models based on water quality data do not account for this increased loading unless samples were collected during rainfall or a spill event and analyzed, and the data were then entered into the model database. Models that account for point sources can easily account for the increased loading by including the spill as an input parameter.
- Documented application to beach and shellfish closure. This factor describes the ability of the model to predict the water quality condition surrounding swimming and shellfish areas. Models can be used as water quality predictive tools and as a basis for decisionmaking. For example, several communities use rainfall models, and the New York City metropolitan area uses the Regional Bypass Model (discussed later in this chapter). These models have proven to be effective tools to protect people from exposure to pathogens following rainfall events or sewage spills.
- Ease of use. The level of user experience is an important factor in determining whether a model is easy to use. Some complex models require a great deal of training and experience; simple methods require only a conceptual understanding of the processes, and results can be readily obtained.
- Input data requirements. Input data requirements are a function of a model's complexity. In general, complex models require more specific and complex input data than simple models. Some of these data might not be readily available, and acquiring such data might require expending resources. Therefore, the objective of the model application can be very important in this step.
- Calibration requirements. Decision making and management alternatives based on modeling results require that the model outcome be acceptable and reliable. Not all models can be calibrated. Models that simulate water quality conditions are calibrated against in-stream monitoring stations. Simple models such as the rainfall alert curves are continuously updated to provide accurate results. This is done by continually updating the model's database.
- Pollutant routing. Pollutant routing addresses how a model deals with the fate and transformation of pollutants. Simpler models might not include processes that describe pollutant transformation. More complex models vary in their description of the processes. The range can be from a gross or a net estimate of the process to a detailed mechanism of the process. The focus is on bacterial processes. In general, most environmental models use the first-order decay rate to represent microbial die-off rate.
- Kinetics of pathogen decay. The survival of pathogens (and pathogen indicators) in the environment is influenced by many variables, such as age of the fecal deposit, temperature, sunlight, pH, soil type, salinity, and moisture conditions. In general, the death rate of pathogens can be estimated as a first-order rate, which is incorporated into water quality models.
Predictive models are effective tools to supplement actual sampling. It is important, however, to consider that models do not provide perfect predictions of actual conditions but instead provide estimates of current conditions. A public health manager should account for inaccuracies in models when making decisions related to public health.
2. Predictive Methods Currently Used by Beach Managers
Two approaches were used to identify the predictive tools currently in use by local agencies. First, EPA's National Health Protection Survey of Beaches was used to identify local agencies that currently base their beach advisories on water quality model prediction. Those agencies were contacted regarding the types of models they use and information about the extent of use, model development, and availability.
The second approach was a review of literature and information from previous EPA programs. This approach included reviewing the models and guidelines provided in the CWA section 301(h) program, identifying tools used in the Total Maximum Daily Load (TMDL) program, and reviewing other EPA publications that relate to water quality modeling. The beach closure predictive tools identified were characterized based on modeling or prediction application techniques and on modeling components.
The tools currently in use by local and state agencies vary in their complexity and approach to minimizing exposure to pathogens. The cities of Milwaukee, Wisconsin, and Stamford, Connecticut, and the Delaware Department of Natural Resources and Environmental Control (DNREC) used regression analysis to relate rainfall to pathogen concentration. Models developed using this approach are site-specific because they are derived from locally observed water quality and rainfall data.
Simulation of water quality conditions under a variety of scenarios of untreated or partially treated sewage also can be used. Comparison of the simulated water quality conditions to the established criteria can serve as the basis for a beach closure. The metropolitan Boston area in Massachusetts is undertaking such a project. A predictive model that can predict water quality conditions resulting from bypasses of sewage at preselected locations was developed for the New York-New Jersey Harbor. Beaches surrounding the discharge locations are closed whenever the predicted pathogen concentrations exceed the water quality criteria.
Closure of beaches based on water quality modeling is also practiced in Virginia and Washington. Computer models that predict pathogen concentration by simulating the dominant mixing and transport processes in the receiving water range from simple to very complex. The Virginia Department of Health uses a simple mixing and transport model to predict water quality conditions surrounding wastewater treatment plant outfalls. The state of Washington uses a more complex model, CORMIX, to predict water quality conditions surrounding wastewater treatment plant outfalls. Rhode Island is also developing predictive models for its beaches. Review of Potential Modeling Tools and Approaches to Support the Beach Program (USEPA, 1999) provides a detailed description of these tools and their attributes, limitations, data requirements, and availability. A summary of the capabilities and applicability of these models is provided in Table 1.
Model | Combined PS/NPS* | Real Time & Decision Making | Spills | Application to Beach or Shellfish Closure | Ease of Use | Input Data Required | Calib. | Developing Guidelines | Pollutant Routing |
---|---|---|---|---|---|---|---|---|---|
Rainfall-based | xxx | xxx | 0 | xxx | xxx | x | xx | xx | 0 |
Bypass | x(PS) | xxx | xxx | xxx | xxx | xxx | x | xx | xxx |
SMTM | x(PS) | xx | xx | xx | xx | x | x | 0 | x |
PLUMES | x (PS) | x | xx | xx | xx | x | x | x | x |
CORMIX | x (PS) | x | x | x | xx | x | x | x | x |
JPEFDC | xx (NPS/PS) | x | xxx | xxx | x | xxx | xx | x | xxx |
0 = Not Applicable; x = Low applicability; xx = Medium applicability; xxx = High applicability; * = Point Source/Nonpoint Source |
3. Rainfall-Based Alert Curves
—3.1. Objectives
The objective of a rainfall-based alert curve model is to establish a statistical relationship between rainfall events and bacterial indicator concentrations. This relationship can then serve as a management tool for developing operating (advisory and closures) guidelines based on predicted pathogen concentrations that suggest the need to restrict or prohibit contact uses of recreation waters. Several agencies have developed beach operating rules based on analysis of site-specific relationships between rainfall and water quality monitoring data. Delaware (DNREC, 1997) and Connecticut (Kuntz, 1998) have successfully used this approach (USEPA, 1999).
—3.2. Benefits
The use of rainfall-based alert curves are highly recommended as predictive tools to determine the need for beach advisories or closings. They are recommended because of their simplicity, ease of development and use, economic feasibility, and virtually instantaneous run time. A great advantage of rainfall-based alert curves is that they can be easily translated to decision logic that a beach manager can use without prior advanced training or a high level of technical skill.
—3.3. Limitations
It is important to update these models with changes in watershed or weather pattern. Weather patterns are typically cyclical, so predictive models must reflect this variance or acknowledge this limitation. For example, rainfall-based alert curves may not be appropriate for use along the arid southern California coast because of an "impact lag" effect where discharges from storm water outfalls can continue for several weeks following substantial rain events.
—3.4. Overview of Rainfall-Based Alert Curves Technical Approach
Rainfall-based alert curves are developed in three phases: collecting data, analyzing data (linking the rainfall events to bacterial indicators), and developing operating rules for advisories or closings of recreational waters. Although EPA is currently supporting continued efforts in research and development of these techniques, the Agency recommends that state, tribal, and local beach managers consider developing scientifically based and easy-to-use site-specific decision rules based on the technical approaches summarized below:
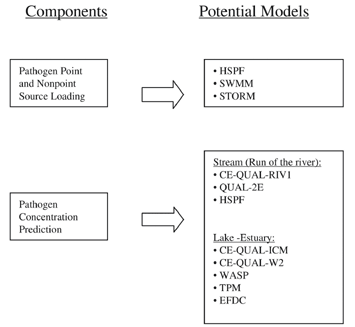
Figure 1. Predictive Tool Summary
- Rainfall-based models are site-specific, and their development requires relatively large sets of monitoring data for both rainfall and water quality. The overall relationship can be described by a statistical regression/estimation model. Depending on the number of rainfall stations considered and the number of rainfall characteristics (amount, duration, lag time), the relationship might require a more complex multiple-regression model. Because of the statistical nature of these types of models, they cannot distinguish between point sources and nonpoint sources of pathogens and do not explicitly incorporate advection, transport, and decay processes. Also, because their use is limited to assisting in the development of decision rules for advisories and closings of recreational waters, they do not attempt to provide the spatial and vertical distribution of pathogens.
- Frequency of exceedance analysis is another rainfall-based method that can be used to develop rainfall-based alert curves. An exceedance is defined as any time the observed pathogen concentration exceeds the action level, such as the state water quality standard, specified by a responsible agency. The objective of this method is to determine the minimum amount of rainfall that causes the pathogen concentration to exceed the action level. This amount can be determined by dividing cumulative rainfall amounts over a period of 24 hours or more into segments that range from no rainfall to an upper limit that is representative of the rainfall record, types of storms, and season. For each rainfall amount category, the observed pathogen concentration or the geometric mean of multiple samples is compared to the action level.
- After establishing a relationship between rainfall amounts and pathogen concentrations, developing decision rules for advisories and closings is the next step. An advisory or closing threshold is determined based on the least amount of rainfall that would result in an exceedance of the action level. This method applies to situations where historical rainfall data and water quality records exist. Decision rules also should be developed to include seasonal variation in rainfall.
—3.5. Case Studies
Rainfall-based alert curves based on regression analysis have been used for preemptive beach closures in Milwaukee, Wisconsin; Stamford, Connecticut; Sussex County, Delaware; and the Boston, Massachusetts, area. In the cases of the city of Milwaukee, city of Stamford, and the Delaware Department of Natural Resources and Environmental Control, the approach taken was regression analysis to relate rainfall to pathogen concentration. Models developed based on this approach are site-specific because they are derived from locally observed water quality and rainfall data as well as beach location/configuration relative to pathogen sources.4. Other Predictive Tools to Supplement Sampling
—Supplemental Sampling
The overall objective of beach closure predictive tools is to minimize the population's exposure to pathogens. The tools currently in use by local agencies vary in their complexity and approach to minimizing exposure but are generally simple, reliable tools. Figure K-1 shows other predictive tools that can be used to determine the need for a beach closing. The listed models are divided into two categories-watershed pathogen loading models and pathogen concentration prediction models. The latter category is divided into two additional groups to reflect the waterbody types: (1) rivers and streams and (2) lakes and estuaries. Currently, there are no readily available models that address the coastal nearshore environment; therefore, surf zone models are not included in this appendix.
Pathogen Loading Estimates
Watershed loading models that can be used to estimate pathogen loadings to receiving waters are presented in Table 2. Three considerations are taken into account in the table-real-time prediction, source type, and land use type.
Model Type | Model Name | Real-time Prediction | Source Type | Land Use Type | ||||
---|---|---|---|---|---|---|---|---|
Data Needs | Processing Time | PS | NPS | CSO | Urban | Rural | ||
Watershed-scale loading | HSPF: Hydrological Simulation Program-Fortran | x | x | xx | x | x | xxx | |
SWMM: Storm Water Management Model | x | x | x | x | xx | xx | x | |
STORM: Storage, Treatment, Overflow, Runoff, Model | x | x | x | x | x | xx | ||
x = Low data requirements/applicability; xx = Medium data requirements/applicability; xxx = High data requirements/applicability. |
Potential sources of pathogens include point sources (including CSOs) and nonpoint sources. Models differ in their ability to account for these various source types. Models that simulate nonpoint sources are capable of describing the pathogen buildup processes during dry weather and washoff processes related to rainfall-generated runoff. Accounting for various land uses is very important in estimating nonpoint source loadings because the processes of buildup and washoff are land-use-specific. CSO loading is a function of the hydraulic routing and the storage capacity in the publicly owned treatment works, consisting of the treatment plant and collection system. Therefore, a model's ability to deal with the complex land uses in the watershed is an important factor in model selection and applicability. The key loading models suited for real-time prediction summarized in Table 2 are briefly described above.
HSPF: Hydrological Simulation Program-Fortran. HSPF is a comprehensive watershed-scale model developed by EPA. The model uses continuous simulation of water balance and pollutant buildup and washoff processes to generate time series of runoff flow rates, as well as pollutant concentration at any given point in the watershed. Runoff from both urban and rural areas can be simulated using HSPF; however, simulation of CSOs is not possible. Because of the comprehensive nature of the model, data requirements for HSPF are extensive and using this model requires highly trained personnel.
SWMM: Storm Water Management Model. SWMM is a comprehensive watershed-scale model developed by EPA. It can be used to model several types of pollutants on either a continuous or storm event basis. Simulation of mixed land uses is possible using SWMM, but the model's capabilities are limited for rural areas. SWMM can simulate loadings from CSOs. The model requires both intensive data input and a special effort for validation and calibration. The output of the model is time series of flow, storage, and contaminant concentrations at any point in the watershed.
STORM: Storage, Treatment, Overflow, Runoff Model. STORM is a watershed loading model developed by the U.S. Army Corps of Engineers for continuous simulation of runoff quantity and quality. The model was primarily designed for modeling storm water runoff from urban areas, but it also can simulate combined sewer systems. It requires relatively moderate to high calibration and input data. The simulation output is hourly hydrographs and pollutographs.
Pathogen Concentration Prediction
Loading models, depending on the simulation type, provide estimates of either the total water and pollutant loading or a time series loading of water and pollutants. Pathogen concentration prediction is the process of describing the response of the waterbody to pollutant loadings, flows, and ambient conditions. Because the response is specific to the waterbody, different types of models are required for accurate simulation, as shown in Table 3. The models are divided into two categories on the table-rivers and streams, and lakes and estuaries.
Rivers and Streams. Prediction of pathogen concentration in rivers and streams is dominated by the processes of advection and dispersion and the bacterial indicator degradation. One-, two-, and three-dimensional models have been developed to describe these processes, as shown in table K-3. Waterbody type and data availability are the two most important factors that determine model applicability. For most small and shallow rivers, one-dimensional models are sufficient to simulate the waterbody's response to pathogen loading. For large and deep rivers and streams, however, the one-dimensional approach falls short of describing the processes of advection and dispersion. Assumptions that the pathogen concentration is uniform both vertically and laterally are no longer valid. In such cases two- or three-dimensional models that include a description of the hydrodynamics are used. The river and stream models summarized in Table 3 are briefly described below.
Model Name | Time-Relevant Prediction | Waterbody Type | ||
---|---|---|---|---|
Data Needs | Processing Time | Rivers and Streams | Lakes and Estuaries | |
HSPF: Hydrological Simulation Program-Fortran | xx | x | x | N/A |
CE-QUAL-RIV1: Hydrodynamic and Water Quality Model for Streams | xx | xx | x | N/A |
CE-QUAL-ICM: A Three-Dimensional, Time-Variable, Integrated-Compartment Eutrophication Model | xxx | xxx | x | xx |
CE-QUAL-W2: A Two-Dimensional, Laterally Averaged, Hydrodynamic and Water Quality Model | xxx | xx | xx | x |
WASP5: Water Quality Analysis Simulation Program | xx | xx | xx | xx |
EFDC: Environmental Fluid Dynamics Computer Code | xx | xx | xx | xx |
QUAL2E: Enhanced Stream Water Quality Model | x | x | x | N/A |
TPM: Tidal Prism Model | x | x | N/A | x |
x = Low applicability; xx = Medium applicability; xxx = High applicability. |
HSPF: Hydrological Simulation Program-Fortran. HSPF is a comprehensive watershed-scale model developed by EPA. The receiving water component allows dynamic simulation of one-dimensional stream channels, and several hydrodynamic routing options are available. The model output is time series of runoff flow rate, as well as pollutant concentration at any given point in the watershed. Because of the model's comprehensive nature, the data requirements for HSPF are extensive and running the model requires highly trained personnel.
CE-QUAL-RIV1: Hydrodynamic and Water Quality Model for Streams. CE-QUAL-RIV1 is a dynamic, one-dimensional model for rivers and estuaries consisting of two codes-one for hydraulic routing and another for dynamic water quality simulation. CE-QUAL-RIV1 allows simulation of unsteady flow of branched river systems. The input data requirements include the river geometry, boundary conditions, initial in-stream and inflow boundary water quality concentrations, and meteorological data. The model predicts time-varying concentrations of water quality constituents.
Lakes and Estuaries. Predicting the response of lakes and estuaries to pathogen loading requires an understanding of the hydrodynamic processes. Shallow lakes can be simulated as a simplified, completely mixed system with an inflow stream and an outflow stream. However, simulating deep lakes or estuaries with multiple inflows and outflows that are affected by tidal cycles is not a simple task. Pathogen concentration prediction is dominated by the processes of advection and dispersion, and these processes are affected by the tidal flow. The size of the lake or the estuary, the net freshwater flow, and wind conditions are some of the factors that determine the applicability of the models. The lake and estuary models summarized in table K-3 are briefly described below.
WASP5: Water Quality Analysis Simulation Program. WASP5 is a general-purpose modeling system for assessing the fate and transport of pollutants in surface water. The model can be applied in one, two, or three dimensions and can be linked to other hydrodynamic models. WASP5 simulates the time-varying processes of advection and dispersion while considering point and nonpoint source loadings and boundary exchange. The waterbody to be simulated is divided into a series of completely mixed segments, and the loads, boundary concentrations, and initial concentrations must be specified for each state variable.
CE-QUAL-ICM: A Three-Dimensional Time-Variable Integrated-Compartment Eutrophication Model. CE-QUAL-ICM is a dynamic water quality model that can be applied to most waterbodies in one, two, or three dimensions. The model can be coupled with three-dimensional hydrodynamic and benthic-sediment model components. CE-QUAL-ICM predicts time-varying concentrations of water quality constituents. The input requirements for the model include 140 parameters to specify the kinetic interactions, initial and boundary conditions, and geometric data to define the waterbody to be simulated. Model use might require significant expertise in aquatic biology and chemistry.
EFDC: Environmental Fluid Dynamics Computer Code. EFDC is a general three-dimensional hydrodynamic model developed by Hamrick (1992). EFDC is applicable to rivers, lakes, reservoirs, estuaries, wetlands, and coastal regions where complex water circulation, mixing, and transport conditions are present. EFDC must be linked to a water quality model to predict the receiving water quality conditions. HEM-3D is a three-dimensional hydrodynamic eutrophication model that was developed by integrating EFDC with a water quality model. Considerable technical expertise in hydrodynamics and eutrophication processes is required to use the EFDC model.
CE-QUAL-W2: A Two-Dimensional, Laterally Averaged Hydrodynamic and Water Quality Model. CE-QUAL-W2 is a hydrodynamic water quality model that can be applied to most waterbodies in one dimension or laterally averaged in two dimensions. The model is suited for simulating long, narrow waterbodies like reservoirs and long estuaries, where stratification might occur. The model application is flexible because the constituents are arranged in four levels of complexity. Also, the water quality and hydrodynamic routines are directly coupled, allowing for more frequent updating of the water quality routines. This feature can reduce the computational burden for complex systems. The input requirements for CE-QUAL-W2 include geometric data to define the waterbody, specific initial boundary conditions, and specification of approximately 60 coefficients for the simulation of water quality.
QUAL2E: The Enhanced Stream Water Quality Model. QUAL2E is a steady-state receiving water model. The basic equation used in QUAL2E is the one-dimensional advective-dispersive mass transport equation. Although the model assumes a steady-state flow, it allows simulation of diurnal variations in meteorological inputs. The input requirements of QUAL2E include the stream reach physical representation and the chemical and biological properties for each reach.
TPM: Tidal Prism Model. TPM is a steady-state receiving water quality model applicable only to small coastal basins. In such locations the tidal cycles dominate the mixing and transport of pollutants. The model assumes that the tide rises and falls simultaneously throughout the waterbody and that the system is in hydrodynamic equilibrium. Two types of input data are required to run TPM. The geometric data that define the system being simulated are the returning ratio, initial concentration, and boundary conditions. The physical data required are the water temperature, reaction rate, point and nonpoint sources, and initial boundary conditions for water quality parameters modeled.
5. References
- DNREC. 1997. Swimming (Primary Body Contact) Water Quality Attainability for Priority Watersheds in Sussex County. Delaware Department of Natural Resources and Environmental Control, Dover, DE.
- Kuntz, J.E. 1998. Non-point Sources of Bacteria at Beaches. City of Stamford Health Department, Stamford, CT.
- USEPA. 1999. Review of Potential Modeling Tools and Approaches to Support the BEACH Program. EPA 823/R-99-002. U.S. Environmental Protection Agency, Office of Science and Technology, Washington, DC.
- Hamrick, J.M. 1992. A Three-dimensional Environmental Fluid Dynamics Computer Code: Theoretical and Computational Aspects. The College of William and Mary, Virginia Institute of Marine Science, Gloucester, VA. Special Report 317.