Annual Spray Drift Review
Office of Pesticide Programs
Environmental Fate and Effects Division
1.0 PURPOSE OF THE PANEL
The Environmental Fate and Effects Division (EFED) of EPA's Office of Pesticide Programs (OPP) wishes to consult with the FIFRA Scientific Advisory Panel (SAP) concerning the scientific validity of the Spray Drift Task Force (SDTF) aerial spray field data and the correlated laboratory and wind tunnel data on physical properties and atomization. OPP and EPA's Office of Research and Development (ORD) also wish to present to the SAP the AgDRIFT model, which simulates downwind deposition of pesticide material following spray application. The ultimate aim of this inquiry is to determine the suitability of the SDTF aerial database and AgDRIFT for regulatory use.
This presentation to the Scientific Advisory Panel is the latest in a series of peer reviews to which the SDTF aerial spray drift data and AgDRIFT model have been subjected. The most recent peer review occurred at the September, 1997 SDTF Data Review Workshop, which centered around reviews of the SDTF database and drift model by a panel of experts from academia, other government agencies, and contracted consultants. OPP believes that such a rigorous review of the Task Force's work will ensure that these valuable tools are used properly for regulatory purposes. A summary of the Workshop proceedings is included in the package for the SAP.
2.0 INTRODUCTION AND SCOPE
The Spray Drift Task Force (SDTF), a consortium of approximately 40 pesticide registrants, has developed a database of field, laboratory, wind tunnel, and related studies designed to provide the necessary relationships for predicting pesticide spray droplet-size spectra and off-target spray drift deposition (amounts vs. distance) under a broad range of application scenarios. Results of the SDTF studies are intended to provide a generic database for fulfilling EPA spray drift data requirements to support the registration of hundreds of pesticide products, and ultimately to provide a quantitative measure of the potential environmental exposure of nontarget organisms to a chemical of concern.
In field trials, the SDTF has sprayed by air (rotary and fixed wing aircraft); ground, including mist blowers or airblast; and chemigation. The Panel is convened here to evaluate aerial spray results only. Under consideration are those pesticide ingredients which are aerially sprayed on target areas as relatively dilute tank mixtures (e.g., emulsions, suspensions) or homogeneous solutions in which another component, usually water or an oil, is the predominant component and carrier. Excluded are granules (which are not sprayed), very highly concentrated suspensions, and invert emulsions/suspensions. Furthermore, because spray drift is sometimes confused with volatilization or other forms of secondary transport, it should be clearly understood that the SDTF work was intended to address primary deposition which occurs within seconds or minutes after application, not secondary movement which is often chemical specific and which may occur over indefinitely long periods.
To achieve their purpose of establishing a generic database, the SDTF investigated the influence of test substances (spray tank contents), application factors (equipment and operating conditions), and meteorological conditions on droplet size and spray drift deposition. For the aerial field studies the SDTF used three different aircraft and a range of droplet sizes. As part of the generic approach, a working hypothesis to be tested was that spray drift was essentially independent of the chemical identity of a pesticide active ingredient; any specific effects would be manifested through the collective physical properties of the entire contents of the tank mix and their influence on the droplet-size distribution.
3.0 BACKGROUND AND RATIONALE FOR THE SDTF WORK
Knowledge of the extent of off-target spray drift deposition resulting from pesticide application is important because of the potential for unintended exposure of nontarget organisms to toxic substances and associated ecological effects. Therefore, predicting the pattern of spray drift (deposition vs. distance) is indispensable for risk assessment and regulatory action. To ascertain such information, the EPA currently may require spray drift studies (droplet-size spectra and field drift) to support registration and reregistration of a pesticide for aerial or ground application methods.
EPA and the registrant community realized that conducting separate field drift studies on all pesticide formulations and product uses which have spray applications is technically and economically impractical. Even if a small subset of application variables (such as nozzle type; pressure; vehicle type, speed, and release height) and meteorological variables (such as wind, relative humidity) for a given formulation could be tested in the field, extrapolation to other situations and formulations would not be possible unless relationships among physical, chemical, application, and weather variables had been previously established experimentally. In other words, as a practical matter, acquiring a limited set of spray drift field studies for a particular pesticide has little general predictive value without correlation or reference to an existing, extensive database.
With this in mind, a group of pesticide registrants formed the SDTF in 1990 to develop a generic approach by which the number of spray drift studies required to support pesticide registrations and reregistrations could be reduced. The SDTF hypothesized from existing data that: 1) droplet-size spectra, 2) aircraft and operating conditions, and 3) meteorological conditions were the primary factors governing spray drift. The SDTF posited further that droplet size-spectra correlated most with a) laboratory measureable liquid physical properties (e.g., surface tension, viscosity) of spray tank contents and b) application apparatus and operating conditions, not the specific chemical or physical properties of the pure pesticide active ingredient. Finally, the SDTF assumed that :
1) measurements of droplet size in a wind tunnel to simulate application conditions are representative of droplet sizes under field conditions, and
2) their study design could cover a sufficiently wide range of test substances and application conditions to represent typical aerial spray practices.
With these hypotheses, the SDTF hoped to establish a scientifically sound, generic database. This resource could then perhaps be used, with the aid of simulation modeling, for the prediction of drift deposition under a variety of application scenarios and environmental conditions.
The generic approach, if validated, has the benefit of reducing the number of required studies conducted, increasing predictability, and permitting better focus on the principal factors influencing spray drift. If successful, results will enable registrants to fulfill necessary data requirements for hundreds of pesticide products with fewer, if any, chemical specific studies, and greater data reliability.
On this basis, in 1992 and 1993 the SDTF conducted field studies to measure off-target aerial spray deposition using a fixed wing aircraft (Cessna AG Husky), a helicopter (Wasp), and a fixed wing turbine-powered aircraft (Air Tractor 502). The studies were designed to measure downwind deposition using varying application parameters and meteorological conditions. The field data were complemented by extensive laboratory physical property and atomization droplet-size spectrum data for pesticide test substances and surrogates. The large collection of physical properties and atomization data included, as a small subset, the test substances and application parameters used in the field.
Another part of the program is the Cooperative Research and Development Agreement (CRADA) with EPA's Office of Research and Development (ORD), which has produced the AgDRIFT spray drift model in order to better predict downwind drift. This model, which is a modification of the previous drift model AGDISP, allows for simulation of a broad-range of application practices and interactions. The completed field studies are being used to aid in model validation.
Although the SDTF field studies cover drift from ground, orchard airblast, and chemigation applications, at this time OPP wishes to consult with the SAP only on the aerial field studies, the correlated physical property and atomization studies, and the aerial portion of the AgDRIFT model.
In addition, EPA/OPP believes that regulatory use of the aerial field studies should be limited to the deposition data at this time. It is not clear how data from low-volume air samplers or vertical alpha-cellulose collectors apply to ecological risk assessments. Moreover, some reviewers noted that the vertical alpha-cellulose collectors may not be efficient collectors of airborne droplets. Also, Kirk (1997) indicated that the set-up which the SDTF used for the low-volume air samplers may not be appropriate for estimating inhalation exposure.
EPA/OPP has arranged for a rigorous peer review of these aerial field studies and supporting lab data. In addition to EPA/OPP review, EPA/ORD, Environment Canada, USDA's Agricultural Research Service, California EPA's Department of Pesticide Regulation, and three individual scientists under contract to OPP have reviewed these data. As the conclusion of this peer review process, EPA/OPP would like the SAP to provide further insight on the scientific validity of the
4.0 SDTF TEST METHODOLOGY
4.1 Field Studies
This section was extracted from Bird and Perry (1996) which has been sent to SAP panelists separately.
In 1992 and 1993, the SDTF conducted three sets of aerial drift field studies. The first study, designated as F92-008 and conducted during July, 1992, in the Texas panhandle (Plainview), was selected to collect data in a hot, dry climate with relatively high winds. A cool-season study (F93-015) was conducted at the same site in April, 1993. The third series of trials (F93-017), selected to collect data in a hot, dry climate, was performed in the Rio Grande Valley of south Texas (Raymondville) during July, 1993. The descriptions of the individual field trials and results are in three submission reports (Johnson, 1994; Johnson 1995b; Johnson, 1995c).
The basic series of trials consisted of a total of 180 field tests applied in pairs (90 pairs) with each pair consisting of a "standard" treatment and a "variable" treatment. The application variables, such as the spray release height, spray volume, formulation and nozzle configuration, were held constant for all "standard" treatments. The "variable" treatments were systematically altered to measure the extremes of normal application practices. The test variables included application rate, droplet size, release height, boom length, airplane speed, and physical properties of the test liquids. For rapid reference and an overview of variables tested in these trials, test variables are summarized in Table II-1 from Bird and Perry (1996). Forty-five "variable" treatments were tested with replicate applications for each treatment pair. In many cases replicates were done several days apart. Data in this table were extracted from the three individual trial reports along with droplet spectra for specific nozzles and test substances, field test flight speeds, and angle of release from atomization study reports (Hewitt 1995a, 1995b).
The "standard" treatments and the "variable" treatments were applied in close succession using different tracers to reduce differences due to meteorological conditions. The purpose of this test design was to provide a method to compare numerous tests over an extended period of time and to evaluate the confounded effects of meteorological conditions. Covariate analysis of the "standard" treatments was used to adjust the "variable" treatments statistically to account for differences in the meteorological conditions during the test period. The 90 replicates for the "standard" treatment provided enough data for a statistical evaluation of meteorological effects as well.
At the Plainview site, the pesticides were applied to a level field of mowed grass (10-15 cm height). At Raymondville, the applications were made over rough-disked grain sorghum stubble mowed to 25 cm. Trial numbers 17-2 and 17-3 at Raymondville were applied over a cotton canopy at the green boll stage, averaging 104 cm in height. The length of the application path for all trials was 610 - 670 m with four parallel lines sprayed 13.7 m apart. Collection stations were established on three parallel lines 15 m apart, centered on and perpendicular to the length of the application zone with downwind stations placed 8, 15, 23, 30, 46, 61, 91, 137, 183, 305, 550 and 792 m from the downwind edge of the application zone. The general field layout at all sites is illustrated in Figure II.1 from Bird and Perry (1996).
Table II-1 from Bird and Perry (1996)
No. | Average Flow (l/ha) | Air 1 Speed | App 2 Height | Boom 3 Length | Nozzle Type (0) | Tracer4 (ml/l) | Carrier | Additives4 | VMD | %<105µ | %<141µ |
Standard Treatments Co-Applied with all Variable Treatment | |||||||||||
1 | 28 | N | L | 68 | D6-46(45) | 8.2-D | Water | P1,MO | 238 | 9.8 | 17.1 |
Standard Treatments Applied at Plainview, TX during the Summer of 1992 | |||||||||||
8.2 | 2.8 | N | L | 68 | 8002(90) | 79.3-M | Oil | MN1 | 160 | 35.3 | 45.1 |
8-3 | 2.8 | N | H | 68 | 8002(90) | 79.3M | Oil | MN1 | 160 | 35.3 | 45.1 |
8-4 | 15 | N | L | 68 | D6-46(45) | 15.1-M | Water | MN2,P1 | 173 | 17.6 | 33.8 |
8-5 | 13 | N | H | 68 | D4-46(45) | 15.1-M | Water | MN2,P1 | 173 | 17.6 | 33.8 |
8-6 | 28 | S | H | 68 | D6-46(45) | 6.6-M | Water | MN3,P1 | 263 | 7.8 | 15.0 |
8-7 | 63 | N | L | 68 | D8-46(0) | 2.5M | Water | P,MN4 | 340 | 2.0 | 6.0 |
8-8 | 65 | N | H | 68 | D8-46(0) | 2.5-M | Water | P1,MN4 | 546 | 0.7 | 2.1 |
8-9 | 70 | N | L | 68 | D8-SS(0) | 2.5-M | Water | P1,MN4 | 546 | 0.7 | 2.1 |
8-10 | 67 | N | L | 68 | D8-SS(0) | 2.5-M | Water | P1,MN4 | 546 | 0.7 | 2.1 |
8-11 | 67 | N | L | 68 | D8-SS(0) | 2.5-M | Water | P1,MN4 | 546 | 0.7 | 2.1 |
8-12 | 29 | N | L | 68 | D6-46(45) | 6.6-M | Water | MN3,P1 | 263 | 7.8 | 15.0 |
8-13 | 36 | F | L | 68 | D6-46(45) | 5.0-M | Water | P1,MN5 | 163 | 22.6 | 38.8 |
8-14 | 68 | F | L | 68 | D8-SS(0) | 2.5-M | Water | P1,MN4 | 413 | 3.2 | 6.5 |
8-15 | 30 | N | L | 68 | D6-46(45) | 6.6-M | Water | P2,MN3, MEK |
178 | 18.9 | 33.8 |
8-16 | 30 | N | L | 68 | D6-46(45) | 6.6-M | Water | P2,MN3,E,S | 318 | 13.8 | 20.3 |
No. | Average Flow (1/ha) |
Air 1 Speed | App 2 Height | Boom 3 Length | Nozzle Type (9) | Tracer 4 (ml/l) | Carrier | Additives4 | VMD | % < 105µ |
% < 141µ |
8-17 | 33 | N | L | 68 | D6-46(45) | 6.6-M | Water | P2,MN3, E,G |
256 | 8.5 | 15.3 |
8-18 | 35 | N | L | 68 | D6-46(45) | 6.6-M | Water | P2,MN3,E G,S |
325 | 12.8 | 19.1 |
8-19 | 30 | N | L | 68 | D6-46(45) | 6.6-M | Water | P2,MN3 | 235 | 12.4 | 20.6 |
Variable Treatments applied at Planview, TX during the Spring of 1993 (SDTF Study F93-0015) | |||||||||||
15-2 | 12 | F | L | 68 | D4-45(45) | 15.1-M | Water | P1 | 107 | 49.2 | 69.9 |
15-3 | 35 | F | L | 68 | D6-46(45) | 5.0-M | Water | P1 | 163 | 22.6 | 38.8 |
15-4 | 72 | F | L | 68 | D8-SS(0) | 2.5-M | Water | P1 | 413 | 3.2 | 6.5 |
15-5 | 16 | N | L | 68 | D4-45(45) | 15.1-M | Water | P1 | 173 | 17.6 | 33.8 |
15-6 | 16 | N | L | 68 | D4-45(45) | 15.1-M | Water | P1 | 173 | 17.6 | 33.8 |
15-7 | 14 | N | L | 68 | D4-45(45) | 15.1-M | Water | P1 | 173 | 17.6 | 33.8 |
15-8 | 74 | N | L | 68 | D8-SS(0) | 2.5-M | Water | P1 | 546 | 0.7 | 2.1 |
15-9 | 69 | N | H | 68 | D8-SS(0) | 2.5-M | Water | P1 | 546 | 0.7 | 2.1 |
15-10 | 73 | N | L | 68 | D8-SS(0) | 2.5-M | Water | P1 | 546 | 0.7 | 2.1 |
15-11 | 30 | N | L | 68 | D6-46(46) | 6.6-M | Water | P1 | 263 | 7.8 | 15.0 |
15-12 | 32 | N | L | 68 | D6-46(45) | 66.1-M | Water | P1 | 359 | 17.8 | 31.2 |
15-13 | 72 | N | L | 68 | D8-SS(0) | 2.5-M | Water | P1 | 546 | 0.7 | 2.1 |
No. | Average Flow (l/ha) | Air 1 Speed | App 2 Height | Boom 3 Length | Nozzle Type (0) | Tracer4 (ml/l) | Carrier | Additives4 | VMD | %<105µ | %<141µ |
Variable Treatments Applied at Raymondville, TX during the Spring of 1993 (SDTF Study F93-0015) | |||||||||||
17-2 | 31 | N | L | 68 | D6-46(45) | 6.6-M | Water | P1 | 263 | 7.8 | 15.0 |
17-3 | 30 | N | L | 68 | D6-46(45) | 6.6-M | Water | P1 | 263 | 7.8 | 15.0 |
17-4 | 12 | S | VL | - | 8003 | 15.1M | Water | P1 | 332 | 2.0 | 6.4 |
17-5 | 25 | S | VL | - | D6-46(45) | 6.6-M | Water | P1 | 339 | 2.1 | 6.2 |
17-6 | 26 | S | MH | - | D4-46(45) | 6.6-M | Water | P1 | 339 | 2.1 | 6.2 |
17-7 | 57 | S | VL | - | D6-SS(0) | 2.5-M | Water | P1 | 811 | 0.1 | 0.2 |
17-8 | 56 | S | MH | - | D6-SS(0) | 2.5-M | Water | P1 | 811 | 0.1 | 0.2 |
17-9 | 74 | N | L | 68 | D6-SS(0) | 2.5-M | Water | P1 | 546 | 0.7 | 2.1 |
17-10 | 14 | N | L | 68 | D6-46(45) | 15.1-M | Water | P1 | 173 | 17.6 | 33.8 |
17-11 | 30 | N | L | 68 | D6-46(45) | 6.6-M | Water | P1 | 263 | 7.8 | 15.0 |
17-12 | 30 | N | L | 68 | D6-46(45) | 75-Sv5 | Water | P1 | 200 | 16.3 | 26.6 |
17-13 | 30 | N | L | 68 | D6-46(45) | 30-O5 | Water | P1 | 241 | 10.8 | 18.8 |
17-14 | 3.2 | N | L | 68 | 8002(90) | 79.3-M | Oil | - | 160 | 35.3 | 45.1 |
17-15 | 5.1 | N | L | 68 | 8003(30) | 39..6-M | Oil | - | 178 | 28.6 | 40.4 |
17-16 | 77 | N | L | 68 | D8-SS(0) | 2.5-M | Water | P1 | 546 | 0.7 | 2.1 |
2 VL-1.6-1.8m;L-2.2-2.9m;MH-4.0-5.4m;H-6.9- 9.3
3 %of wingspan;
4 D-Diazinon AG500;M-Malathion 57EC; Sv-Svein 80S;O-Orthene 75S;P1-15 ml/l phospate buffer w/3.4% Na2HPO4 and 6.6% KH2PO4;P2-60 ml/l phosphate buffer w/3.4% Na2HPO4 and 6.6% KH2PO4; MO - 1.5 g/l (NH4)2Mo2O7-4H2O;MN1 - 30 g/l MnSO4 -H2O; MN2 - 5.6 g/l MnSO4 - H2O; MN3 - 2.5 g/l MnSO4 - H2O; MN4 - 0.94 g/l MnSO4 - H2O; MN5 - 1.9 g/gal MnSO4 - H2O; MEK -185 ml/l methyl ethyl ketone; E - 12 g/l EDTA; G - 119 m/l glycerol; S - 35.4 ml/l Staput;
5 tracer concentration is in g/L.
Horizontal alpha-cellulose cards (type GR512 made by Proctor & Gamble) cut into four 15.2 x 15.2 cm pieces to facilitate handling, were placed at canopy level at all collection stations to measure ground deposition. Diazinon was used as the tracer for all "standard" treatments; malathion was the tracer for most "variable" treatments. In a few cases, alternative active ingredients (ai), including carbaryl and acephate, were used as the tracer. For most cases, the target nominal rate was 0.01 g/m2of tracer. In a few cases, with the alternate tracers, the much higher full application rate from the product label was applied. The nominal application rate is defined as the total mass of material applied divided by the area of the application zone.
Samples were collected within 30 minutes of application, sealed in clear Kapak bags, placed on dry ice, and taken to a freezer trailer for storage. For each trial, two sets (weathered and unweathered) of field spikes for each tracer were used to evaluate the amount of material potentially lost from collectors during sampling. One set was spiked from the sample tank mix and immediately frozen (unweathered); the second was "weathered" by being exposed to ambient conditions during the sampling and collector pick-up time period.
In addition to the cards, low volume air samplers containing polyurethane foam (PUF) were maintained at a height of 1.52 m at 30, 91 and 305 m downwind of the application zone. The PUF samplers were calibrated to collect an air flow rate of 2 L/min and were designed to measure drift that was potentially inhalable. The polyurethane plugs were cylindrical with a diameter of 2.54 cm and a depth of 2.54 cm, fitted into a cylindrical tube positioned with the tube pointed down. Vertical alpha cellulose collector plates were placed alongside the PUF samplers to provide a measure of flux through the vertical plane and as a surrogate for potential dermal exposure.
During the Raymondville trials, vertical string collectors 6 m in length were suspended from metal support towers starting 30 cm from the ground at 3, 30 and 91 m downwind of the application zone. Only data for malathion residues from the "variable" treatments are available; diazinon was found to be unstable on the string based on field spike data.
On-site meteorological measurements, including wind speed, wind direction and air temperature at 0.3, 1.8, 3.05 and 9.1 m above ground, and relative humidity and pressure at 1.8 m, were recorded throughout each trial.
Equipment
Aircraft type and speed, operational pressure, nozzle type, and nozzle placement on the boom are major equipment variables that should be included in an evaluation of the appropriateness of equipment testing during the SDTF trials. Prior to the field trials, the SDTF sponsored a survey of aerial applicators (L92-001, Kidd, 1994), along with modeling sensitivity studies, to identify key variables controlling offsite drift and to assist in the selection of application variables for the field trials. The survey was distributed to members of the National Agricultural Aviation Association (NAAA), the aerial applicators' professional association. The survey represents responses on the order of 40% of the active applicators in this trade association.
Three separate aircraft were used in the SDTF field trials-- (1) a fixed-wing, piston-powered aircraft (Cessna Ag Husky); (2) a fixed-wing turbine-powered aircraft (Air Tractor 502); and (3) a Wasp helicopter. The two fixed-wing aircraft are both popular representatives of their respective horsepower class for aircraft used in agricultural spraying. Although slower airspeed (piston-powered) aircraft were reported more frequently in the survey, a trend toward increasing use of the faster, turbine-powered aircraft was also noted. No information on the distribution of helicopter models was given in the survey report. While the selection of the aircraft for the SDTF studies generally appeared consistent with usage at the time of the 1992 survey, several reviewers expressed concern about the representativeness of these aircraft, especially the Wasp helicopter, with today's usage.
The field trials encompassed a wide range of nozzles, application volumes, and nozzle angles. According to the applicator survey, a medium volume (2-5 gpa) application, a 450 release angle, disc-core nozzle type, a water-based carrier, a moderate airspeed (75-120 mph), and a 25-35 psi boom pressure were the most frequently selected application categories. The standard case application is consistent with these common application conditions. Although the ranges of the variable applications utilized in the SDTF field studies do not cover all application conditions (e.g. the number of nozzle types is somewhat limited), a significant fraction of application conditions are covered along with a suitable range of values for equipment parameters identified as important in the sensitivity study (Teske, 1992).
Carriers/Formulations
Water-based carriers were used in all but four of the variable application trails (which were oil). The applicator survey indicated that the overwhelming majority of applications were water-based. Surfactants, and to a lesser extent, drift control agents were primary additives listed by applicators. The bulk of the studies were performed using a low rate of ai in a buffered solution. A limited selection of additives, including methyl-ethyl ketone, glycerol, and Sta-Put, were applied in a series of trials and found to have little impact on either atomization or drift. Two full-formulation applications of Orthene 75S and Sevin 80S were included for comparative purposes. No significant differences were observed from these applications and use of the tracer. The SDTF is preparing an extensive database of atomization data covering a wider range of formulations and additives. Accepting the generic assumptions discussed previously, one can extrapolate the atomization results from this expanded database to off-site drift potential for a wider range of formulations than were tested directly.
Meteorological Conditions
Meteorological conditions at the three sets of field trials are summarized in Bird and Perry (1996), Tables III.1-III.3, for the standard case applications. With few exceptions, the descriptive statistics for the standard cases closely follow those of the entire data set. Moderate wind speeds and neutral to unstable atmospheric conditions dominated in all three studies. The wind-speed range, from about 1 to 8.0 m/s, coincides with minimum wind speed currently recommended on generic pesticide labeling, but includes many cases considerably higher than the maximum recommended wind speed. In fact, the median speed of 4.68 m/s is just over the recommended maximum of 4.47 m/s (10 mph). Therefore, about half of the cases can be considered as having relatively high wind speeds. This explains the preponderance of near-neutral stability as shown by the Richardson number median value of 0-0.06 (slightly unstable to neutral). Temperature and relative humidity both cover a wide range (temperature from freezing to 350 C; relative humidity from 7 to 94%).
To complement the standard-condition summary of tables III-1 through III-3, Appendix A of Bird and Perry (1996) contains a series of histograms showing the distribution (for the 82 standard cases) of seven meteorological variables: wind speed, relative humidity, wet-bulb depression, Richardson number, angular difference between wind and instrument line, air temperature, and solar radiation. Distributions of each variable are shown across all standard cases and for each of the three field studies.
Studies F92-008 and F93-017 were conducted during warm to hot conditions (temperatures between 20 and 350 C), while F93-015 included much cooler conditions with temperatures mostly between 0 and 200 C. Relative humidity values in studies
Tables III 1-3 from Bird and Perry (1996)
size="-1"> Table III.1 Summary
Statistics of Spray Period Wind Speeds (at 2 meters) during
SDTF Field Trials; Standard (diazinon) Cases Only. |
|||||
Study | No. of Trials | Mean (m/sec) | Median (m/sec) | Minimum (m/sec) | Maximum (m/sec) |
Plainview '92 (08) | 28 | 4.12 | 4.10 | 1.65 | 6.91 |
Plainview '93 (15) | 24 | 4.91 | 5.13 | 2.76 | 8.04 |
Raymondville '93 (17) | 30 | 4.89 | 4.94 | 1.69 | 6.60 |
Overall | 82 | 24.6/56.6 | 27.2/57.0 | 0.4/7.3 | 35.1/93.7 |
size="-1">Table III.2 Summary Statistics for Temperature and Relative Humidity (at 2 meters) during SDRF Field Trials; Standard (diazinon) Cases Only. | |||||
Study |
No. of Trials |
Mean (°C/%) |
Median (°C/%) |
Minimum (°C/%) |
Maximum (°C/%) |
Plainview '92 (08) | 28 | 27.6/63.5 | 27.5/62.7 | 21.5/35.6 | 33.7/92.0 |
Plainview '93 (15) | 24 | 13.4/39.7 | 12.6/35.7 | 0.4/7.3 | 28.2/90.9 |
Raymondville '93 (17) | 30 | 30.8/63.6 | 31.5/58.3 | 24.3/43.2 | 34.1/93.7 |
Overall | 82 | 24.6/56.6 | 27.2/57.0 | 0.4/7.3 | 35.1/93.7 |
size="-1">Table III.3 Summary Statistics for Spray Period Richardson Number during SDTF Field Trials; Standard (diazinon) Cases Only | |||||
Study |
No. of Trials |
Mean | Median | Minimum | Maximum |
Plainview '92 (08) | 28 | -0.07 | -0.05 | -0.26 | 0.03 |
Plainview '93 (15) | 24 | -0.07 | -0.06 | -0.22 | -0.05 |
Raymondville '93 (17) | 30 | -0.08 | -0.07 | -0.21 | 0.02 |
Overall | 82 | -0.08 | -0.06 | -0.26 | 0.05 |
F92-008 and F93-017 were greater than 50% in greater than three-quarters of the cases. In contrast, F93-015 has relative humidities below 50% in over 65% of the cases. While the temperature and relative humidity suggest that F92-015 is a "cool-dry" study, the wet-bulb depression (which is related to temperature and relative humidity) is actually a better indicator of evaporation rate of spray droplets. Although study F92-015 does contain includes a few cases with large wet-bulb depressions (very dry air), the entire overall distribution is not significantly different from F93-008. In all three studies, about half of the wet bulb depression values are less than 60 C, indicating generally humid cases.
Wind speeds for all three studies generally ranged from 1 to 8 m/s with 92-08 having slightly lower values on average. Although the deposition values were corrected for wind direction deviation from the perpendicular downwind line of collectors, study 93-17 showed a greater number of cases with deviations of less than 10 degrees. This simply means the winds were more cooperative in Raymondville. Study F92-008 contained several trials with excessive wind angles (>30%) that were outside the limits of the protocol and where significant chances of missing the most distant downwind collectors existed.
Another important meteorological parameter that influences spray drift is atmospheric stability. The task force estimated stability by calculating the Richardson number (Ri). In all three experiments (based on Ri estimates), the stability was limited to a range of near-neutral to moderately unstable, with no cases of moderate or highly stable conditions. It is not uncommon for moderately stable conditions to exist both early in the morning, before solar radiation has a chance to "burn-off" the nighttime stable layer or late in the evening near dusk where (for clear skies) stable layers can set up quickly. These test data do not reflect the latter situations. Although applications during stable (specifically "inversion" or very stable) conditions pose high drift potential, early morning applications where near-ground, slightly-to-moderately stable conditions occur frequently and are difficult to avoid.
The Richardson number depends on the vertical temperature and wind speed gradient over the layer of interest. Since these gradients are small over shallow layers near the ground, Ri can be difficult to estimate accurately. For high mean wind speeds, Ri reduces to low values (neutral conditions). Figure III-1 shows the relationship between Ri and wind speed for the standard cases. With a few exceptions, the atmosphere becomes more neutral (smaller absolute Ri) with increasing wind speed. The five cases with positive Ri (slightly stable) do not fit the trend because they all occurred with the combination of relatively low wind speeds and extremely low solar radiation values. Figure III-2 shows the relationship between Ri and solar radiation. Again, there is a reasonable trend with more neutral cases being associated with low radiation and generally unstable cases associated with higher radiation levels. There are a few anomalous cases with nearly identical wind speed, humidity, temperature, and Ri, but solar radiation values varied by a factor of almost four. This highlights the problems sometimes encountered with estimating Ri.
In summary, the meteorological conditions cover a fairly wide range and represent conditions expected to be present in most field applications. The major exception is that the SDTF database contains a majority of cases that are unstable or near neutral; there are no experiments conducted in moderate-to-strongly stable conditions.
Figure II-1 from Brid and Perry (1996)
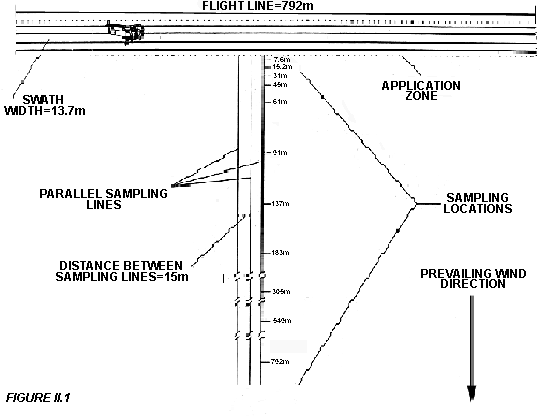
Summary of Variable Treatments for the SDTF Field Study |
|
CATEGORY OF VARIABLE |
NUMBER OF TREATMENTS |
Medium speed aircraft, across three fields, at two volume rates | size="1" >6 |
Three aircraft with high volume rate | size="1" >4 |
Three aircraft at medium volume rate | size="1" >4 |
Three aircraft at low volume rate | size="1" >4 |
Three volume rates at low aircraft speed | size="1" >3 |
Four volume rates at medium aircraft speed | size="1" >12 |
Three volume rates at high aircraft speed | size="1" >3 |
Three boom heights at low volume rate and medium aircraft speed | size="1" >5 |
Same, but at high volume rate | size="1" >5 |
Two boom heights at medium volume rate and medium aircraft speed | size="1" >5 |
Two boom heights at high volume rate, medium aircraft speed and two nozzle types | size="1" >5 |
Two boom heights at two volume rates and low aircraft speed | size="1" >4 |
Two boom widths | size="1" >2 |
Three evaporation rates and a range of VMD of the substances | size="1" >5 |
Two spray rates with two swath numbers | size="1" >4-5 |
Canopy vs Bare Soil | size="1" >2 |
Stable air applications vs unstable air applications | size="1" >5 |
Three swath adjustments | size="1" >3 |
4.2 Physical Properties and Atomization Tests
The purpose of the laboratory tests was to measure droplet-size spectra produced by test fluids with different physical properties as they emerged from representative spray nozzles operating under variable conditions and configurations. Droplet sizes were measured in a wind tunnel to simulate field conditions. In addition to nozzle type, other application variables were nozzle orifice size, liquid pressure at the nozzle tip, nozzle angle relative to the airstream, and airstream velocity in the wind tunnel.
Test substance physical properties which were examined included dynamic surface tension, shear viscosity, and extensional viscosity. Test substances containing active ingredients were prepared from commercial pesticide products representing a wide range of active ingredients and pesticide formulation types. Surrogate test substances encompassed a range of physical properties which fell approximately within a cubic matrix where the x-axis would correspond to dynamic surface tension at 20 msec with a nominal range of 25 to 73 dyne/cm, the y-axis to a shear viscosity with a nominal range of 1 to 52.3 centipoise, and the z-axis to a maximum extensional viscosity with a nominal range of 77 to 2351 centipoise.
Five key laboratory studies were performed and are described below. Two studies were physical property studies and three were atomization studies. These include: (1) a physical property study to generate dynamic surface tension data on test substances used in the atomization and field studies; (2) a physical property study to generate shear and extensional viscosity data on test substances used in the atomization and field studies; (3) a range-finding atomization study; (4) an atomization study to provide droplet-size spectrum data for surrogate test substances; and (5) an atomization study to provide droplet-size spectrum data for 52 pesticide tank mixtures at their maximum label concentration. Atomization studies were performed using Malvern laser diffraction particle size analyzers, sometimes complemented by a Sympatec instrument for droplet sizes too large for the Malvern analyzer.
Dynamic Surface Tension Measurements of SDTF Test Substances
A physical properties study, P93-005, was performed to generate dynamic surface tension data on test substances used in the atomization and field studies. Surface tension is a measure of the minimum energy required for liquid atomization into droplets. The SDTF acknowledged the dynamics of the atomization process by including the time-dependence of surface tension. The surface tension of newly formed test substance-air interfaces was measured on a time scale of 1-20 msec to approximate that of atomization. Measurements on such a rapid scale are necessary to quantify the ability of the surfactants in a tank mix to lower the surface tension during the atomization process. The ability of surfactants to lower surface tension is controlled by the rate at which they can diffuse to the newly-formed surface. The dynamic surface tension/surface age (time) profiles revealed either constant surface tension with surface age or surface tension which decreases with increasing surface age. The latter behavior is more characteristic of agricultural test substances used in this study and of solutions containing moderate concentrations of surfactant.
Shear and Extensional Viscosity Measurements on SDTF Test Substances
A physical property study, P93-004, was performed to generate shear and extensional viscosity data on test substances used in the atomization and field studies. Test substances included non-Newtonian fluids, that is, fluids whose shear viscosity varies with shear rate. (Non-Newtonian systems are more prevalent in concentrated products, especially if the product contains particulates in excess of 5-10% by weight or if polymers of high molecular weight {i.e., greater than 100,000} are present). Since shear viscosity varies with shear rate, the shear viscosity of test substances was measured for a range of shear rates from 100 to 8,000 per second.
Whereas elasticity is a measure of the resistance of a mass to extension and stretching, extensional viscosity is the analogous property applied to liquids/fluids. High extensional viscosity tends to cause a liquid to become resistant to being stretched, thereby affecting atomization. Extension rates applied to test substances ranged from 1,000 to 20,000 per second.
Range-finding Study
Study A91-001, a range-finding study, was performed to determine the droplet-size spectra produced from test substances with a wide range of physical properties. Ten test substances with a range of dynamic surface tension, shear viscosity, and maximum extensional viscosity were atomized in the New Mexico State University wind tunnel and the droplet size spectra were measured. The test substances were selected based on their expected physical properties, which were intended to span the ranges occurring in most agricultural tank mixes. The test substances were atomized through two nozzle tips selected to represent the most common nozzle types used in spraying, and because the droplet size spectra produced by these nozzles typically contained a relatively high proportion of small droplets. A wider range of nozzle tip types and orifice sizes were examined in a subsequent atomization study, A92-003. The test substances were sprayed at relatively medium and high liquid pressures (i.e., 30 and 60 psi) under low and high air shear conditions (i.e., airstream velocities of 110, 50 and 9 mph and nozzle angles of 90 and 0 degrees relative to the airstream).
Statistically Designed Atomization Study for Surrogate Test Substances
Study A92-003 was statistically designed to provide droplet-size spectrum data for surrogate test substances with a wide range of application conditions and a wide range of physical properties, including dynamic surface tension, shear viscosity, and extensional viscosity. These surrogate test substances contained no pesticide active ingredients. The application scenarios comprised different combinations of the following nozzle and test substance application variables: nozzle types, nozzle tips, air velocities, liquid pressures, and nozzle angles (see table in Question 6 below). Fifteen different nozzles were used with three orifice sizes from each of five nozzle types. The five nozzle types included flat fan, disc-core, deflector, full cone, and solid stream. The flat fan spray nozzle produces flat fan spray plumes, the disc-core nozzle produces hollow cone plumes, the deflector nozzle produces sheets of liquid, the full cone nozzle produces full cone plumes, and the solid stream nozzle produces straight jets. Air velocities examined include 40, 60, 80, 120, and 160 mph. The SDTF also examined eleven liquid pressures: 10, 20, 30. 35, 40, 45, 50, 60, 80, 100, and 120 psi. Six nozzle angles relative to the airstream were examined: 0, 15, 30, 45, 60, and 90 degrees. Ranges of physical properties examined in this study were those defined by the range-finding study, A91-001.
It was not considered feasible or necessary to test every combination of each nozzle variable, test substance application variable, and test substance physical property considered in this study. Instead, a statistical "1/2 replicate of a 26 factorial, 'star' design with a center point, replicated twice" was used to cover the range of experimental variables.
The six main effect variables examined in the current design are apparently:
1) pressure
2) nozzle angle
3) relative airstream velocity
4) dynamic surface tension
5) Trouton ratio (derived from maximum extensional viscosity and shear viscosity)
6) shear viscosity.
Atomization of Tank Mixes Containing Active Ingredients
Study A92-004 measured the droplet-size distribution from atomizing a range of test substances containing active ingredients under some of the same nozzle configuration and application conditions used with surrogate test substances in Study A92-003. The treatments were selected to provide very high, moderate, and low shear atomization under both ground and aerial application conditions with the three most common nozzle types (i.e., flat fan, disc-core, and deflector) at a medium flow rate. Test substances encompassed a wide range of active ingredients, pesticide formulation types, and pesticide functionalities. Pesticide functionalities included herbicides, insecticides, fungicides, and other products. The principal formulation types examined included suspension concentrate, dry flowable, wettable powder, emulsifiable concentrate, soluble liquid, water soluble bag, microencapsulated, and concentrated aqueous solution. High and low concentration active ingredient formulations were selected for testing from each formulation type. Tank mix adjuvants were also added to some formulations to represent the effect of that practice on test substance physical properties. The adjuvants examined were Silwet L-77TM, a surfactant known to produce low surface tension and Sta-PutTM, a polyacrylamide polymer reported to produce a high extensional viscosity.
Regression equations were developed to determine the characteristics of the lower to middle portion of the droplet-size spectrum. The four multiple linear regression equations for predicting droplet sizes of less than 50 µm, 141 µm, 220 µm and volume median diameter (Dv0.5) were derived from application parameters and physical properties. The regression equations were based on 1470 atomizations of surrogate test substances and 382 atomizations of commercial based tank mixes of mostly the dilute aqueous type with occasional oil-based tank mixes.
5.0 THE AgDRIFT MODEL
A copy of the AgDRIFT model and supporting documentation have been provided to the SAP separately. The following is a summary of AgDRIFT.
The AgDRIFT model was developed to provide predictive support for matrix categories or real-world situations that could not be represented from the field data.
AgDRIFT, which was developed under the CRADA between EPA/ORD and the SDTF, "predicts the motion of spray material released from aircraft . . . toward a prediction of spray drift" (EPA, 1997). AgDRIFT, which was derived from the earlier drift model AGDISP, is divided into three tiers:
Tier I
The first-tier assessment is a screen that allows the user to evaluate: (1) off-site deposition out to 1,000 ft downwind for a series of atomization regimes, and (2) the potential effectiveness of buffer zones for mitigation. Current generic label instructions do not specify specific droplet regimes for application, but call for the largest droplet sizes that will provide sufficient control and coverage. Therefore, this tier uses preset model runs which incorporate recommended upper limits consistent with good application practices as input values, providing a "reasonable but conservative" estimate of spray drift. Some of these assumptions include:
- 10 mph winds perpendicular to flight path
- neutral stability
- short grassed fields
- moderate evaporation scenario
- application height of 10 ft
- Air Tractor AT-401 aircraft
- no nozzles beyond 3/4 of wingspan
- aqueous carrier
The preset model runs of the Tier I aerial analysis use droplet regimes from British Crop Protection Council, which are defined as very fine, fine, medium, coarse and very coarse. Each threshhold is defined by a single droplet size spectrum. The correspondence between atomization category and spray application rate was based on SDTF wind tunnel studies A92-003 and A92-004.
Model simulations were performed to define downwind drift along these threshholds, thereby developing a series of deposition regimes corresponding to the spray classification regimes. If no spray quality specification is included on the product label, then the fine/medium spray threshhold curve is to be used.
Tier I model curves are also available for ground sprayer and airblast sprayer assessments. Drift estimates by this screen are based solely on SDTF field data from 1995. Ground sprayer simulations allow the user to choose between high or low boom height. Orchard airblast simulations are provided for normal, dense or sparse tree spacings, as well as for individual crops. Higher tier simulations are not available for these two application methods. The ground sprayer and orchard airblast capabilities of AgDRIFT are not being considered by this SAP.
The model allows the user to choose between the various droplet sizes and the size of the receiving water body (including a standard EPA pond scenario). If the concentration of the pesticide in the water body from drift exceeds a level of concern, the user can specify buffer zones of various widths to evaluate how wide a buffer zone might be necessary to mitigate the concern.
Tier II
Tier II of the AgDRIFT model requires an increased knowledge of the application equipment, environment, site and product. The user can change many of the model variables within fixed, preset limits. Table II of the AgDRIFT documentation (provided separately) lists most of these variables and the ranges recognized for Tier II AgDRIFT simulations.
The user can also choose between carrier types (water or oil), and aircraft (Cessna Ag Husky, Wasp helicopter or Air Tractor AT-502). Droplet size distributions can be defined by the user, chosen from the Tier I set or a droplet size distribution the SDTF library included with the model, or generated using the DropKick regression model described below.
Tier III
The AgDRIFT manual states that "Tier III modeling provides the free access to all model variables and assumes the user is an application specialist with a thorough understanding of the atmospheric transport of small particles." Variables that can be changed only in Tier III include the wind direction, which need not be perpendicular to the direction of application, and the effective roughness height of the ground cover.
DropKick Model
AgDRIFT derives the droplet-size distribution either from the SDTF atomization library or from the DropKick model, which also allows access to the SDTF nozzle and physical properties libraries. The DropKick component predicts a droplet-size spectrum from nozzle type, application and spray mix physical property data. It uses a set of regression equations to estimate four drop-size class parameters coupled with an interpolation routine to generate a drop-size distribution that can be used to simulate deposition by AgDRIFT. These regression equations were developed from and tested for a very limited set of nozzles as described in the SDTF draft Integrated Atomization/Physical Property Report, I95-003. Those studies consisted primarily of dilute aqueous tank mixes, but included some oil-based data.
These equations have not been tested with data sets which were collected independently of the regression equation development. The SDTF intends to use several hundred additional atomization data sets which were not used in the development of the regression model to verify this atomization/physical property modeling approach. Until that extended verification assessment is performed, the DropKick option within AgDRIFT should be limited to use in an experimental mode. Alternatives to the current regression approach to an atomization model, such as development of algorithms with a neural network and development of equations for drop-size distribution are currently being pursued by members of the SDTF modeling subcommittee.
The CRADA modeling subcommittee will provide a model evaluation document to the SAP as supplemental information before the Panel convenes on December 11, 1997. The document will consist of six sections:
(1) a review of existing modeling technologies;
(2) overview of the field trials and brief discussion of uncertainties associated with the field trial data;
(3) an overview of AgDRIFT, AgDRIFT input requirements and calculation of inputs for simulation of field trials;
(4) comparison and statistical analysis of residuals between deposition measurements and model output;
(5) model vs field study sensitivity; and
(6) summary and conclusions.
In general the model and field comparisons will be presented in the context of an exploratory data analysis rather than rigorous model building or hypothesis tests. The bulk of the analysis has centered around an evaluation of model/field "residuals," or more precisely, the ratio of AgDRIFT predictions to measured deposition. The analysis will also highlight the responses of model and field study results with regard to potential drift management options, including restrictions on drop-size classes, mandating buffer zones between fields and sensitive areas, and limitations on wind speed during application.
6.0 THE PEER REVIEW PROCESS
OPP determined from the beginning that a wide-ranging peer review process would be the most effective way to ensure the best possible scientific assessment of the data.
Independent Scientific Reviews
The aerial field data and atomization and physical properties studies have been reviewed by OPP and external experts, including EPA's Office of Research and Development (ORD), USDA's Agricultural Research Service, California Department of Pesticide Regulation, and Environment Canada. Also, OPP contracted with three independent scientists to review the data. Each independent organization or individual prepared a written assessment of the aerial field data and supporting atomization and physical properties studies. These very important reports have been submitted to the SAP as "Reviewer Comments--Spray Drift Task Force Data Review Workshop."
Workshop
This peer review of the SDTF's aerial field and laboratory studies culminated in the two-day SDTF Data Review Workshop held in September, 1997, that included over 50 industry, government, and university scientists. The workshop included summary reviews of the SDTF data by the independent scientific reviewers, a demonstration of the AgDRIFT model, breakout sections to discuss key scientific issues related to the field and laboratory studies and their integration, and a wrap-up session to discuss the findings of the breakout groups.
7.0 ISSUES IDENTIFIED BY REVIEWERS OF THE SDTF AERIAL DATABASE
EFED asked reviewers of the SDTF data to focus their comments on a series of common topics to facilitate discussion at the workshop. These can be summarized by the following questions:
1) Are the reports scientifically sound in terms of study design, analytical methods and data collection, statistical analyses and interpretation?
2) Do the data support the generic approach used by the SDTF, i.e. is drift independent of the chemistry of the active ingredient?
3) How do the physical properties and atomization studies on spray mixtures relate to the field studies?
4) What are the limitations of the data set for predicting potential exposure of nontarget organisms to pesticide drift?
5) What factors most influence off-target spray drift of pesticides?
6) To what extent can the data be related to drift that might result from typical aerial pesticide applications?
Question 1: Are the reports scientifically sound in terms of study design, analytical methods and data collection, statistical analyses and interpretation?
Atomization and Physical Properties Studies
All reviewers generally agreed that the data are scientifically sound. The atomization and physical properties studies included a broad range of application equipment, operating conditions and active ingredients, and generated highly reproducible droplet-size spectra. However, most reviewers emphasized that a more detailed description of the experimental design and statistical analyses of data are needed. Included should be a clear listing of the six "independent" or main effect variables used in the analysis, their ranges, and possible interactions or confounding effects. Detailed analysis of variance tables are therefore necessary.
The stepwise regression analysis as presented was not compelling. The SDTF should provide the rationale for adding additional variables, and the criteria for adding or removing terms based on R2 to determine the goodness of fit. The validity and effect of the inverse cosine transformation is unclear and may be biasing droplet-size spectra and the influence of volume median diameter. Correlation effects (multicollinearity) among the 15 variables selected could lead to unstable, inflated, or unrealistic regression coefficients. Therefore, a table of specifications for regression coefficients is needed. As a "reality" check, this table should be compared in directionality with predictions from fundamental physics. Another reality check could be based on splitting the data and comparing results of separate regressions. An explanation is needed for terms with zero degrees of freedom. More direct alternative methods, such as standardized regression, should be explored or used for corroboration. In any case, standard errors and confidence limits associated with the various factors and any final empirical equations need to be clearly established. These issues have been discussed with the SDTF, which has agreed to address them.
The SDTF should provide the rationale for choosing a dynamic surface tension at 20 msec, a shear viscosity at a shearing rate of 8,000/sec, and an extentional viscosity at an extension rate of 20,000/sec for model development, rather than, say, a mean or median value over a range of values. Although in the analysis which the SDTF presented these parameters accounted for most of the physical property effects on droplet-size spectrum, it would be desirable to know what other measures or physical properties were examined and what were their effects.
The SDTF's reason for developing regression equations to estimate various cumulative characteristics of the droplet-size spectrum and the median droplet diameter, instead of the entire distribution, should be clarified.
Field Studies
While the overall field study design was judged by all reviewers to be scientifically sound, several aspects of the studies may limit the extent to which the database may be applied.
Atmospheric Stability
Several reviewers noted that since only moderately stable air was encountered, the data do not shed new light on drift potential under stable air or temperature inversions. Open literature data (Thistle, 1996) suggest that drift potential is greater in low-lying temperature inversions.
EPA agrees that information on drift under stable conditions is important, but we believe the database encompasses a sufficiently wide range of environmental conditions to enable its use for most regulatory purposes. Since the movement of fine particles under stable conditions is likely to be unpredictable and attempts to include inversion conditions in the field trials were unsuccessful, the SDTF notes that a different study design might be required to evaluate drift under these conditions. EPA's concern over applications made in temperature inversions is already addressed in label advisory language.
Data Collection and Analysis
The following factors reduce the certainty of the results from far-field collectors:
(1) Lack of confidence intervals around the data
The reviewers agreed that a full description of the statistical analysis and associated confidence intervals for downwind deposition means are essential. Sources of variability in deposition data identified by several reviewers include line-to-line variation, variability in field spike recoveries, variability in tank mix measurements and potential changes in wind direction angle. Understanding the amount of variability in deposition data will facilitate data interpretation, including comparisons of field measurements with AgDRIFT simulations. The SDTF has agreed to address the issue of confidence limits on deposition data (SDTF letter of 10/29/97).
(2) Spike Recoveries For Low Levels
Spike recoveries for low-level concentrations of malathion and diazinon on alpha-cellulose collectors were erratic, increasing the relative degree of error and uncertainty associated with low collection concentrations. Mulchi (1997) observed that the high-spike samples had low variability and nearly 100% recovery. However, recoveries for low spikes varied from 300-400% down to 20-30%. Since spike information is used to correct recovery, the issue of low-level spike problems should be addressed. The SDTF needs to correct the data for spike recovery or otherwise address this issue.
(3) Wind direction angles may miss downwind collectors
As the wind direction angle from perpendicular increased, the likelihood of drift bypassing far-field collectors increased. Such incidences would lead to underestimations of far-field deposition, increasing the uncertainty of these data. As noted earlier, the AgDRIFT model apparently overestimates far-field deposition compared to field-trial results. The degree to which these errors counterbalance each other is uncertain.
(4) Tank Mix Measurements
Mickle (1997) and others note considerable variation in the concentration of spray tank mixtures taken before and after application.
Deposition efficiencies of the collectors
The collectors used in this study demonstrated a wide range in collection efficiency. The greatest uncertainty in deposition data appears in the far-field collectors. Several reviewers noted that collection efficiencies for the various collectors were not provided. This information could help explain the sometimes widely different results between different collectors, and provide a better measure of the uncertainty in the data. The spike recovery irregularities result in greater uncertainty in far-field deposition data. The limitations of the data (or degree of uncertainty) due to the lack of collection efficiency information needs to be defined.
Mass Balance
Although the SDTF attempted a mass balance based on the horizontal deposition collectors, most of the reviewers questioned the usefulness of this approach. The field study design did not account for smaller droplets that moved beyond the last collector and therefore does not allow for a mass balance analysis to be undertaken. Also, Mickle (1997) noted that an insufficient number of horizontal collectors was used in-swath to assess with accuracy the amount of spray which landed on target. Most reviewers agreed that the data is more useful in evaluating near-field rather than far-field deposition.
Statistical Analysis
The SDTF used a covariate analysis to "adjust" the variable treatment data for meteorological variations. However, the reviewers observed that value of the database is in the data itself, not in the attempt to assess differences between treatment means. For this reason, EPA is asking the SDTF to provide the raw deposition data which has not been adjusted by the covariate analysis.
In each of the field treatment pairs, the standard treatment was designed to supply an environmental baseline which, through covariate analysis, could statistically account for the influence of meteorological conditions on drift.
A key assumption of the field study was that by running a standard treatment with each variable application, the effects of different meteorological conditions across treatments could be "removed" through covariate analysis. That is, the results of variable applications could be adjusted to "average" conditions, which were defined by the results of the standard treatments across all applications.
The analysis of covariance approach assumes that a parallel linear relationship exists between the standard (covariate) and the variable treatments. The independent variables of the covariate are fixed and measured without error. There is independence between a value of the standard and the effect of a treatment.
Numerous reviewers (Barry, 1997, EFED, 1997a, Zhang 1997, Mickle, 1997, Mulchi, 1997) pointed to several problems with the design. Because standard and variable measurements could not be taken simultaneously, neither measurement should be considered with respect to environmental conditions. For example, parameters of the wind (speed and direction) could change between the standard treatment line and the variable treatment line, thus defeating the purpose of the analysis of covariance.
Bird and Perry (1996) point out the covariate analysis assumes that the effect of changing wind speed will be the same on large droplet as small droplets, which could explain why the regression generates negative deposition values. They suggest that the covariate correction could over-correct low drift cases and under-correct higher drift cases. They also suggest that the covariate correction approach be redone to allow for the non-linearities of the various application variables as they respond to different meteorological conditions.
Barry (1997) concludes that although the assumptions of covariate analysis do not hold up for these field studies, 1) the 90 standard applications represent a large body of data useful for evaluating the effects of weather on spray drift of pesticides, and 2) the database can be useful in validating the AgDRIFT model.
An alternative statistical analysis by Mickle yielded conclusions similar to the covariate approach; i.e., the three principal factors affecting drift are droplet size, spray release height and wind speed.
Question 2: Do the data support the generic approach used by the SDTF, i.e., is drift independent of the chemistry of the active ingredients?
The general conclusion reached by all reviewers is that the data, as presented, show that primary drift is independent of the chemical identity of the active ingredient (non-volatile), except as may be manifested through physical property effects on the droplet-size spectrum. Droplet size is the only major spray drift factor which the active ingredient could affect. Physical properties and atomization data ostensibly show that it is not necessary to invoke the identity of the active ingredient in order to predict droplet-size spectra. However, firm conclusions can not be established until the gaps in the statistical analyses have been filled.
Bird and Perry (1996) and Mickle (1997) discuss one treatment (08-19) where malathion tracer and carbaryl at full strength were applied together, but do not show the same results. Mickle suggests that this discrepancy might be accounted for by the tank mix uncertainties. Bird suggests there could have been slightly different "micrometeorological" conditions near the two booms used, causing slight differences in deposition in the far field.
Question 3: How do the physical properties and atomization studies on spray mixtures relate to the field studies?
The physical properties and atomization data are related to the field studies through the droplet-size distribution. The limited number of combinations of test substance ingredients and application variables (nozzles, nozzle configurations, spray pressures, spray volumes, airspeed) used in the field studies were contained within the range of the larger set of those used in the physical properties and atomization experiments. Therefore, droplet-size spectra in the field studies are tied to atomization data to the extent that the simulated spray conditions in the wind tunnel represent the field conditions. Hence, if droplet sizes are indeed critical factors influencing drift, then these values are obtainable for use with field data for input to a putative predictive model.
The physical property and atomization studies resulted in the active ingredient-independent development of four multiple linear regression equations for predicting droplet size-spectrum measures (V<50 µ, V<141µ, V<220µ, and DV0.5) from application parameters (such as those related to nozzle type and size, air velocities, liquid pressures, and nozzle angles) and the physical properties of surrogate and commercial tank mixtures (dynamic surface tension, shear viscosity, and extensional viscosity). The SDTF has agreed to provide additional information on the regression equations based on comments from science reviewers.
The field data provided information on spray drift deposition resulting from the spraying of different tank mixes with measured physical properties under different known application conditions.
By comparing the application parameters and conditions in the field study to the measured physical properties in the atomization studies, a droplet-size spectrum of the tank mix in the field study can be estimated. The estimated droplet-size spectrum, in conjunction with meteorological (wind speed and direction; relative humidity, atmospheric stability, etc.) and application factors (aircraft speed and height, etc.) can be correlated with the measured spray drift depositions in the field studies. Such correlations can then be used to develop spray drift models that estimate droplet size spectra and spray drift deposition from the physical properties of the tank mix and non-meteorological and meteorological application conditions.
Field Studies
Droplet-size spectra were not measured directly in the field but were inferred from the wind tunnel simulations. Adjuvants and tank mix additives intended to alter spray mixture physical properties, droplet size, or evaporation rate appeared to have little impact on off-target movement. However, droplet size, as influenced by application parameters (nozzle factors, spray volume, and air speed), was a major factor in drift potential.
The SDTF reported that none of the substances tested had a statistically significant effect on the droplet-size spectrum, although the volume mean diameter (VMD) for the methyl-ethyl ketone treatment was smaller (VMD = 178µ) compared to other treatments (VMD = 235-270µ) with the same equipment set-up. Also, the methyl-ethyl ketone treatment had the highest evaporation rate. Additional evaporation data are being developed .
Aircraft turbulence present in field experiments was not simulated in the wind tunnel studies. This is an area of uncertainty with regard to comparing droplet sizes in wind tunnel atomization studies to those produced in the field studies. The issue of turbulence with respect to the spray release was raised. Zhang and Kuhlman (1997) noted that droplet sizes measured in a wind tunnel may be different from droplet size in the field where variable shear across a boom is present.
The SDTF indicated that it is difficult to measure the possible range of droplet size spectra that could be produced by the enormous variety of air speed and turbulence conditions that might occur at all possible nozzle/wing/boom combinations used in the field. The SDTF data were measured under relatively controlled conditions covering the range of air velocities typical in the field in order to study the effects of application and physical property variables on emission droplet size. The SDTF has agreed to address turbulence in a white paper on wind tunnel simulations of aerial spraying.
Question 4: What are the limitations of the data set for predicting potential exposure of nontarget organisms to pesticide drift?
Deposition data will be used to estimate exposure of nontarget organisms following aerial applications of pesticides. The limitations of the data are outlined under question 1 above. Depositional data may also be used by the OPP Health Effects Division (HED) to estimate surface residues potentially available for post-application human dermal contact; for example, on residential yards adjacent to treated fields. Similarly, the vertical card and string sampler data may be used to estimate human dermal and inhalation exposures, respectively, that might result from accidental direct contact with airborne residues migrating off-site.
Atomization and Physical Properties Studies
Several reviewers (EFED, 1997b, Kirk, 1997) argue that no general conclusions can be drawn from the lab data alone since the lab data could not adequately represent exposure to non-target organisms. However, as spray drift increases, the chance of non-target exposure also increases. Therefore the initial droplet-size spectrum, to the extent reproducible in the lab, does influence exposure to non-target organisms.
Field Studies
Several reviewers (Akesson, 1997, Biermann, 1997) suggest that plants as collectors would allow for a better evaluation of exposure to non-target organisms than horizontal cards. Mulchi (1997) also suggests that the correlation between deposition on horizontal collectors and potential deposition to aquatic or terrestrial surfaces should be undertaken.
Further, the applicability of data from the air samplers or vertical collectors to ecological risk assessments is uncertain. As noted in the discussion for question 1, some reviewers questioned the efficiency of the vertical collectors in capturing airborne droplets. Also, Kirk (1997) indicated that the set-up used for the low-volume air samplers used by the SDTF may not be appropriate for estimating inhalation exposure.
Despite these uncertainties, OPP believes that use of the SDTF field data will add considerable precision to its current parctice of assuming that 5% of the aerial pesticide is deposited 100 feet downwind of the target site.
Question 5: What factors most influence off-target spray drift of pesticides?
Firm conclusions can not be established until the gaps in the statistical analyses have been filled.
Atomization and Physical Properties Studies
The SDTF reported that application or mechanical variables accounted for 82-91% of the variation in the atomization process, while physical properties accounted for 9-18% of the variation. However, under increasing shear conditions (e.g., increasing aircraft speeds), the effects of physical properties appear to increase signficantly.
The three most important physical property factors were the dynamic surface tension and the shear and extensional viscosities. In the A91-001 range finding study on the atomization of surrogate test liquid mixtures, the SDTF concluded that when application parameters were kept constant, the physical property characteristics of the dynamic surface tension at 20 msec, the shear viscosity at a shearing rate of 8,000/sec, and the extensional viscosity at an extension rate of 20,000/sec accounted for 67 to 95% of the variance across different test substances.
The laboratory studies appear to show that:
(1) Active ingredients have little or no effect on the droplet-size spectrum.
(2) Application factors (such as those related to nozzle type and size, air velocities, liquid pressures, and nozzle angles) affect droplet size spectra to a greater extent than the physical properties of surrogate and commercial tank mixtures (dynamic surface tension, shear viscosity, and extensional viscosity) for typical low-shear conditions.
Nevertheless, regression equations for predicting measures of the droplet-size spectrum do include significant primary physical property terms, significant cross-terms (application x property), and significant dimensionless terms (depending upon both application and physical property factors), in addition to significant primary application terms. Consequently, physical properties cannot be ignored in estimating the droplet-size spectrum, especially under high-shear conditions.
Field Studies
Results of the aerial field studies indicate that droplet size, as inferred from wind tunnel simulations, spray boom height, and wind speed are the primary factors influencing downwind depositon.
A stepwise regression of data from the standard treatment suggests that wind speed was the only meteorological factor which which appreciably influenced downwind deposition. Some reviewers commented that secondary factors such as temperature, relative humidity, and solar radiation, could have an effect on drift by affecting droplet size. However, these parameters as measured in the field studies had no statistically-significant effect on drift. In addition, a narrow selection of adjuvants which were intentionally added to alter spray mixture physical properties, droplet size, or evaporation rate had relatively little impact on off-target drift potential. The SDTF has attempted to account for droplet-size decreases with recent enhancements to the AgDRIFT model.
Question 6: To what extent can the data be related to drift that might result from typical aerial pesticide applications?
Atomization and Physical Properties Studies
Droplet-size spectra were determined for tank mixes of 33 active ingredients from commercial pesticide products covering the current major agricultural use categories and formulation types. Excluded were granules (which are not sprayed), high concentrate suspensions, and invert emulsions/suspensions.
Application equipment and operating conditions for the lab and wind tunnel studies included typical settings and "reasonable worst case" settings. Many different nozzle types and sizes were used. However, not all nozzle types were tested, e.g., electrostatic and sonic nozzles. The SDTF data and any resulting model should not (cannot) be applied to nozzles which are based on operating principles not yet tested. For those nozzles tested, angles varied from 0 degrees (straight back) to 90 degrees (straight down). Spray pressures ranged from 10 to 120 psi. Air velocities ranged from 9 to 160 mph.
The regression equations developed by the SDTF for estimating droplet-size spectrum characteristics from application and physical property parameters were based upon 1470 atomizations of surrogate test substances in study A92-003 and 382 atomizations of commercial based tank mixes in study A92-004. The studies concentrated primarily upon dilute aqueous tank mixes, but some oil-based data were also collected.
The surrogate and commercial tank mixes studied reportedly covered physical properties and application parameters as summarized in the table on the following page.
The nozzles mentioned below do not cover a sufficiently broad range of those currently used in aerial applications. However, the SDTF conducted additional studies on numerous additional nozzles (list attached) as provided by manufacturers. These studies have not yet been reviewed by OPP. When reviewed, these additional data will be more representative of the range of contemporary nozzles used in aerial application. Furthermore, the droplet-size distribution data from these nozzles can be used as an independent source for model validation, and perhaps eventually added to the model to increase its statistical power.
SUMMARY OF PHYSICAL PROPERTIES AND APPLICATION PARAMETERS | |
PHYSICAL PROPERTY | RATE OR TYPE |
Dynamic Surface Tension at 20 ms | 25-73 dyne/cm |
Shear Viscosity at Shearing Rate of 8,000/sec | 1-52.3 cP |
Extensional Viscosity at an Extension Rate of 20,000/sec | 77-2351 cP |
Nozzle Types | Flat Fan, Disc-Core, Deflector, Full Cone, Solid Stream |
Nozzle Sizes per Type | 8002, 8006, 8010 (flat fan); D4-23, D6-45, D6-46 (disc core), TK-1, TK-3, TK-5 (full cone), D2-SS, D4-SS, D6-SS (solid stream) |
Air Velocities | 40-160 mph |
Liquid Pressures | 10-120 psi |
Nozzle Angles | 0-90 degrees |
Field Studies
While the overall database appears to cover a broad range of typical application conditions, reviewers noted several factors that may affect the applicability of the data to drift from aerial applications.
Absence of data on drift from stable air and low-lying inversion conditions
As noted in question 1, EPA believes that information on drift under stable conditions is important, but that the database encompasses a sufficiently wide range of environmental conditions to enable its use for most regulatory purposes.
Omission of Some Application Practices
Several reviewers expressed concern that the applicator survey may not be representative of a sufficiently wide range of typical application practices, such as the nozzle use discussed above, because of the small sample size. However, the database should still be valid for those combinations of application practices that produce a droplet-size spectrum represented by the database. This database should only be used for interpolation within the bounds of spectra represented, and not for extrapolation beyond those bounds.
Range of Aircraft Used in Study
Kirk (1997) suggests that aircraft type could be of concern when comparing the field studies to real-world application. The Cessna Ag Husky is the primary aircraft used in the field studies. However, according to a SDTF survey of aerial applications, this aircraft is only used 18% of the time in real-world applications. Kirk says, "the representation of the SDTF standard treatment on current aerial application is further diminished by the fact that the 80 plus % of the aircraft not represented by the standard treatment cover considerbly more than 80 % of the acreage treated by agricultural aircraft."
Although a limited range of fixed-wing aircraft were tested, reviewers agreed that field tests for a wider range of fixed-wing aircraft are not needed. The aircraft type itself is not as critical as the configuration of the application equipment coupled with the aircraft speed. Akesson (1997) and Biermann (1997) indicated that further field tests using other types of helicopters are needed to better characterize the potential for spray drift from rotary aircraft.
Applications to Four Swaths
The field study involved four swath applications per treatment. Full fields or multiple field treatments were not undertaken. In addition, the potential of pesticide loading (multiple passes, pesticide lingering for a few days, volatilization, etc.) was not studied.
OPP believes that the data obtained from a series of systematic experiments using four-swath field applications is a significant improvement over spray-drift data available previously. The four swaths at the downwind edge of a field account for much of the off-target movement of the pesticide. Moreover, drift from full-field applications can be extrapolated mathematically from the four-swath experiments. OPP believes that four-swath studies, when extrapolated mathematically to represent a 20-swath application, is suitable for most regulatory purposes.
Terrain Issues
Several reviewers noted that the database does not capture effects of canopy interception on drift. In addition, some questioned whether the terrain adequately represented typical conditions for aerial applications. The terrain represented in the field studies included level-mowed field grass, rough bareground or grain sorghum stubble, and some trials on cotton.
OPP believes that the SDTF's choice to spray over a standard terrain is acceptable because it would not be practical to field-test a large range of terrain and canopy types. Bare ground and low-cut grass canopies, by limiting canopy interception of spray applications, provide a conservative scenario for measuring spray drift.
8.0 SUMMARY
The Spray Drift Task Force (SDTF), a consortium of approximately 40 pesticide registrants, has developed a database of field, laboratory, wind tunnel, and related studies designed to predict pesticide spray droplet-size spectra and off-target spray drift deposition (amounts vs. distance) under a broad range of application scenarios. Results of the SDTF studies are intended to provide a generic database, i.e. drift is a function of fluid physical properties, equipment factors, and meteorological conditions, not the chemical identity of the pure pesticide active ingredient. These data are intended to be used to fulfill EPA spray drift data requirements to support the registration of hundreds of pesticide products and to provide a quantitative measure of the potential environmental exposure of nontarget organisms to a chemical of concern.
The generic approach, if validated, has the benefit of reducing the number of required studies conducted, increasing predictability, and permitting better focus on the principal factors influencing spray drift. If successful, results will enable registrants to fulfill necessary data requirements for hundreds of pesticide products with fewer chemical specific studies and greater data reliability.
The Environmental Fate and Effects Division (EFED) of the Office of Pesticide Programs (OPP) wishes to consult with the FIFRA Scientific Advisory Panel (SAP) concerning the scientific validity of the SDTF aerial spray field data and the correlated laboratory and wind tunnel data on physical properties and atomization. OPP and EPA's Office of Research and Development (ORD) also wish to present to the SAP the AgDRIFT model, which simulates downwind deposition of pesticide material following spray application. AgDRIFT has been developed by ORD and the SDTF under a Cooperative Research and Development Agreement (CRADA). The ultimate aim of this inquiry is to determine the suitability of the SDTF aerial database and AgDRIFT for regulatory use.
This presentation to the Scientific Advisory Panel is the latest in a series of peer reviews to which the SDTF aerial data have been subjected. The most recent peer review occurred at the September, 1997, SDTF Data Review Workshop, which centered around reviews of the SDTF aerial database by a panel of experts from academia, other government agencies, and contracted consultants. OPP believes that such a rigorous review of the Task Force's work will ensure that these valuable tools are used properly for regulatory purposes. AgDRIFT is currently undergoing ORD peer review. AGDISP, the model from which AgDRIFT was developed, has been presented through peer reviewed journals.
Scientific reviewers of the aerial field data and correlated atomization and physical properties studies identified areas of uncertainty or areas where additional information or analyses are needed. These have been discussed in detail with the SDTF which has agreed to address the issues raised, provide the requested information, and to perform the additional analyses. With this cooperation from the SDTF and with further guidance from the SAP after it has considered the questions and issues raised by ORD and OPP in this document and in their presentations, OPP believes that the SDTF aerial field data will be suitable for regulatory use. Further, ORD and OPP believe that AgDRIFT will then add considerable precision to its current practice of assuming that 5% of an aerially applied pesticide is deposited 100 feet downwind of the target site.
9.0 REFERENCES
Akesson, N. B., 1997. Spray Drift Task Force, Science Peer Review Report. University of California, Davis, CA. Unpublished Report.
Barry, T., 1997. Spray Drift Task Force, Science Peer Review Report. California EPA, Unpublished Report.
Bird, S. L. and S. G. Perry, 1996. Review of Spray Drift Task Force Data Used in Aerial Model Evaluation, Environmental Protection Agency, National Exposure Research Laboratory. EPA Internal Report
EFED, 1997a. Summary and Minutes of the OPP-EFED Spray Drift Workshop, Sep 11-12, 1997. Environmental Protection Agency, Environmental Fate and Effects Division (EFED), Unpublished Report
EFED, 1997b. Responses to Questions Regarding Spray Drift Task Force Aerial Field Trials and Atomization and Physical Properties Studies. Environmental Protection Agency, Environmental Fate and Effects Division (EFED), Unpublished Report
Fox, R. D., 1997. Spray Drift Task Force, Science Peer Review Report. USDA, Agricultural Research Service, Wooster, OH. Unpublished Report.
Hewitt, A. 1995. Atomization Droplet Size Spectra for Spray Drift Test Substances: 1992 Field Trial Conditions SDTF Studies # A92-005/A93-008 (two documents), SDTF, McKenna and Cuneo, Washington D.C.
Johnson, D., 1994. Spray Drift Task Force: 1992 Aerial Field Study in Texas: Malathion, Diazinon: Lab Project Number: F92-008. Unpublished study prepared by Spray Drift Task Force; Agrisearch Inc. and New Mexico State Univ., Dept. of Entomology. 663 p.
Johnson, D., 1995. Spray Drift Task Force: 1993 Cool Season Aerial Field Study in Texas: Lab Project Number: F93-015. Unpublished study prepared by Stewart Agricultural Research Services, Inc. 443 p.
Johnson, D., 1995. Spray Drift Task Force: 1993 Hot, Humid Aerial Field Study in Texas: Lab Project Number: F93-017. Unpublished study prepared by Stewart Agricultural Research Services, Inc. 724 p.
Johnson, D.R., 1995. Drift from Applications with Aerial Sprayers: Integration and Summary of 1992 and 1993 Field Studies. SDTF Report # I94-002, SDTF, McKenna and Cuneo, Washington D.C.
Kirk, B., 1997. Spray Drift Task Force, Science Peer Review Report. USDA-Agriculture Research Service, College Station, Texas, Unpublished Report
Mickle, B., 1997. Spray Drift Task Force, Science Peer Review Report. Atmospheric Environment Service, Environment Canada. Unpublished Report.
Mulchi, C. 1997. Spray Drift Task Force, Science Peer Review Report. University of Maryland, Unpublished Report
Thistle, H.W. 1996. Atmospheric stability and the dispersion of pesticides. Journal of the American Mosquito Control Association 12(2):359-363.)
Zhang, N., 1997. Spray Drift Task Force, Science Peer Review Report. Kansas State University, Unpublished Report